Yes.
Bridge Capture™ and Nicking Loop™ are covered by an extensive patent portfolio. Genomill owns all patents related to these technologies, providing unparalleled freedom in operations, and eliminating any concerns related to intellectual property issues.
Currently there are nine patents across four patent families:
Accurate and massively parallel quantification of nucleic acid; ZL201880055271.3, EP3673081, HK40017021, JP7074978.
Methods for accurate parallel quantification of nucleic acids in dilute or non-purified samples; EP4060049, US11898202.
Highly sensitive methods for accurate parallel quantification of nucleic acids; EP4060050, US11486003.
Methods for accurate parallel detection and quantification of nucleic acids; US11970736.
Bridge Capture™ is a novel approach and methodology when compared to existing amplicon-based and hybrid capture methods. Nicking Loop™ is a completely new way of thinking sequencing libraries in circular format directly sequencable by circular DNA sequencers (Complete Genomics’s DNBSEQ, Element Aviti, PacBio Onso) with being able to access the libraries with database-like functions. Therefore, the IP portfolio around Geno1® technologies are highly differentiated from other solutions.
Yes.
The Bridge Capture™ and Nicking Loop™ have been designed to produce sequencing libraries that are compatible with any NGS platform. This is made possible by the circular libraries which can be sequenced either with short-read or long-read platforms. See FAQ interlinks below for an overview of how the Bridge Capture™ and Nicking Loop™ technology outputs fit to different sequencing platforms.
Yes.
Genomill has developed a panel with 887 probes which targets 123 genes containing 24,236 mutations, from which 1,870 are oncologically relevant mutations (See Figure 1). The panel design is based on the COSMIC database, CMC v.99 (https://cancer.sanger.ac.uk/cosmic).
Genomill’s panel is fully modular, since panel can be split into multiple smaller panels and from these the desired subpanels can be combined back into one bigger panel, still retaining their probe performance.
Yes.
Bridge Capture™ has been demonstrated to reliably detect SNVs, indels, and gene fusions. We also have preliminary results of detecting CNVs. Bridge Capture® can detect mutations associated with any cancer type, given the right panel design.
Nicking Loop™ has been demonstrated with SNVs and due to the shared functions with Bridge Capture™ we assume Nicking Loop™ to handle the same mutation types.
Yes.
The smallest amount of patient cfDNA tested with Bridge Capture™ thus far has been approximately 2000 genomic copies (5 ng). The detection of low allelic fractions benefits from a larger amount of sample used in the workflow.
Yes.
New probes can be introduced into an existing panel when required. Experimental panel validation is mandatory after introduction of new content.
Yes.
Bridge Capture™ workflow permits sample pooling after the initial targeting step without the need for normalization whereas other commercially available technologies usually pool the pre-indexed amplified libraries just before sequencing. This approach ensures that as the number of samples processed in parallel increases, the associated increase in workflow duration, cost, and complexity does not follow a linear trend, offering a distinct advantage in efficiency and scalability. Our pooling approach is currently protected within our patents and undergoing further improvements.
In the Nicking Loop™ workflow the samples can be pooled after introducing and ligating the Nicking Loops.
Yes.
The Bridge Capture™ workflow is highly versatile and can easily be adapted to automated and scalable environments. Recent demonstrations of the workflow were performed with Opentrons OT-2, a popular automation platform for life sciences, where the workflow was completed in a single run.
Bridge Capture™ workflow can be fully automated depending on the chosen automation platform and configuration, encompassing both workflow and library preparation for NGS analysis in the same run. This allows for even greater efficiency and precision in processing large numbers of samples. In addition, the hands-on load time for the automation workflow is brief, taking only approximately 5 minutes to complete.
Yes.
The Nicking Loop™ workflow has been demonstrated with Opentrons OT-2.
No.
After the cfDNA is extracted from the sample, purified material can be used straight for the Bridge Capture™ and Nicking Loop™ workflows. Sample doesn’t need to be diluted, as it is unlikely that amount of cfDNA in sample would saturate the number of probes used in the Bridge Capture™ or the adapters in the Nicking Loop™.
No.
Genomill obtains probe oligomers and other oligonucleotides from well-established commercial providers. Currently, Genomill uses IDT as a commercial provider.
Yes, optionally.
Bridge Capture™ is designed to be compatible with UMIs, which can be particularly useful for ultrasensitive quantitative applications, such as detecting low allele fractions from cfDNA. However, UMIs may not be necessary for the applications where simple presence or absence quantification is required.
Yes.
Amplicon-based techniques are based on multiplexed PCR which has inherent limitations in multiplexing, sequencing evenness and rare target detection. Amplicon-based workflows can be improved by introducing UMIs but this leads to a more complicated workflow.
Bridge Capture™ overcomes these limitations.
Bridge Capture™ provides improvements in scalability, sensitivity and workflow simplicity. Bridge Capture™ is a simple protocol, optionally utilizing UMIs (see FAQ interlinks below), with infinite scalability, high evenness, and high sensitivity for rare variants. This results in improved performance as well as a streamlined, highly cost-efficient protocol.
Yes.
Bridge Capture™ provides improvements in chemistry and workflow costs (see FAQ interlinks below), as well as improvements in speed, and simplicity. The Bridge Capture™ workflow itself is simple and cost-efficient and results in a sequencing library with high target evenness. Since this library is focused on pre-selected loci, even very rare variants can be called with moderate sequencing depth.
In contrast, hybrid capture-based techniques are based on sequencing library preparation workflows and extend them by introducing an additional enrichment step where the desired parts of the sequencing libraries are enriched using capture probes. Hybrid capture techniques permit profiling a large number of mutations from sample material but suffer from expensive and complicated laboratory workflows, sequencing unevenness, high sequencing requirement, and complicated data analysis.
Bridge Capture™ overcomes these limitations and provides a lean, sensitive and cost-efficient alternative to hybrid capture.
Yes.
Bridge Capture™ has advantages in performance, scalability and cost-efficiency in comparison to molecular inversion probes.
Molecular inversion probes are composed of a lengthy single-stranded DNA molecules including linker region and target-specific segments at both ends. This continuous structure involves high probe synthesis costs as well as significant challenges and limitations on the probe design process. Molecular inversion probes are typically amplified using PCR primers specific to the linker sequence, resulting in uneven target amplification and probe cross-reactivity.
In contrast, Bridge Capture™ uses a design featuring short, target-specific probe arms connected by a bridge oligonucleotide (see FAQ interlinks below). This innovative structure significantly lowers the probe synthesis costs, streamlining the design and testing procedures. The modular nature of Bridge Capture™ – with each probe comprising three separate oligonucleotides – facilitates seamless implementation of novel design ideas and modifications on targeting parts, permitting approaches like sample barcoding on targeting, and PCR-free DNA sequencing libraries.
Unlike the conventional PCR amplification of molecular inversion probes using primers specific to the linker sequence, Bridge Capture™ utilizes linear signal amplification. This approach avoids probe cross-reactivity, ensuring highly uniform and reproducible results.
Yes. Optionally
Bridge Capture™ is designed to be compatible with UMIs, which can be particularly useful for ultrasensitive quantitative applications, such as detecting low allele fractions from cfDNA. However, UMIs may not be necessary for the applications where simple presence or absence quantification is required.
With our in-house probe designer, the probes typically work with good evenness (See Figure 1). We have shown that panel evenness can be further improved by adjusting individual probe concentrations.
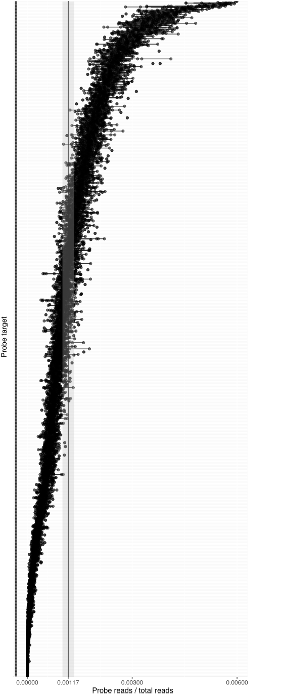
No.
Genomill has developed an efficient and highly scalable probe design method. It is based on a streamlined proprietary in-house algorithm able to design functional probes in minutes. Genomill’s probe production pipeline involves also rigorous experimental testing, ensuring the highest possible quality of each probe used.
Yes.
The performance of Bridge Capture™ was compared against ArcherDX LiquidPlex and AmpliSeq for Illumina Cancer Hotspot Panel v2 with comparable results. All the compared technologies were carried out by a global 3rd party CRO highly experienced with NGS.
Quote from an expert technician in NGS preparations at the CRO: “This is by far the easiest workflow I’ve ever used.”
Yes.
Bridge Capture™ workflow has been validated with two local CROs in early 2023 and with one global-scale CRO / precision diagnostic company in mid-2023.
The UMI sequences are used in the Bridge Capture™ and Nicking Loop™ data processing in error correction. Error correction assumes that a single UMI should be associated with a single sequence, and any deviation from this is a result of an amplification or sequencing error. Taken together with the read count, the UMI sequences permit very accurate quantification of molecular counts with a high signal-to-noise ratio.
In the Bridge Capture™ workflow, the process of circularizing the probe involves several steps. First, a polymerase is introduced to fill the gap between the two probes. The polymerase uses the target sequence as a template to fill in the gap between the probes. As the polymerase extends the sequence, it eventually meets the other probe, and a ligase joins the two probes together. Optionally, a Nicking Loop™ can be introduced for early sample indexing and Nicking Loop™ functionalities.
Furthermore, during the polymerase and ligase reaction, the gap that exists between the probes in the bridge area is also filled, thus ligating the probes together from this end as well. This leads to the formation of a full circular molecule with the bridge still hybridized to it. The bridge sequence is an essential part of the probe design, as it helps to hold the two probes together and maintain the circularized structure of the molecule.
The resulting circularized probe is now ready for amplification through RCA (see FAQ interlinks below) or it can be optionally sequenced directly with a circular-based sequencer like Complete Genomic’s DNBSEQ, Element Aviti or PacBio Onso.
Limited-cycle indexing PCR uses primers with overhangs to introduce the required adapters for the sequencing platform of choice. The PCR is done in limited cycles to minimize the exponential amplification as the main amplification is done in RCA.
RCA is a nucleic acid amplification technique that involves the circularization of an ssDNA template, followed by repeated extension of a DNA polymerase around the circularized molecule, resulting in the formation of long, branched DNA molecules.
In Bridge Capture™ process the RCA is primed from the bridge oligo, which is extended by Phi29 DNA polymerase. As the Phi29 polymerase progresses around the circularized template, it displaces the previously synthesized strand, resulting in the formation of a long, concatemeric DNA molecule. Phi29 is highly processive, and extension results in the formation of many copies of the original circular template, leading to efficient linear amplification of the target sequence.
The resulting concatemeric ssDNA can be processed further for linear sequencing platforms with limited-cycle indexing PCR (see FAQ interlinks below). The concatemeric ssDNA product can also be sequenced with long-read sequencing platforms, such as Oxford Nanopore Technologies.
The probe-bridge constructs are introduced into a hybridization reaction with the sample material. The number of probe-bridge constructs is significantly higher than the sample material to improve the kinetics of the hybridization. Optionally, the targeting can be done with Nicking Loops present, which will be integrated to the circular structure in the GapFill step (see FAQ interlinks below). This enables a very early pooling of samples in the workflow (see FAQ interlinks below).
The standard gap length in Bridge Capture™ is approximately 50 base pairs. This length was chosen to strike a balance between the small size of cfDNA fragments and the number of mutations that can be captured by a single probe pair when the gap length is increased. The longest gap length tested so far is 110 base pairs, while the shortest gap tested was a nick between the probes, which was used to quantify specific molecules.
Probe arms are 20 base pairs long on average. This results in a typical combined footprint of 90 bp for left and right probes together with the gap region.
There is no predefined upper limit for the targets (number of probes) in a panel. So far, our largest panel has contained 887 probes and it worked as expected.
The reproducibility of the read counts reported by Bridge Capture™, determined by replicated sequencing experiments, and indicated as mean-normalized replicate counts, is typically between 0.5x to 2x of the replicate mean (see Figure 1).
The sensitivity is reproducible between replicates and was demonstrated experimentally in validation experimentation done on NovaSeq 6000 (see FAQ interlinks below)
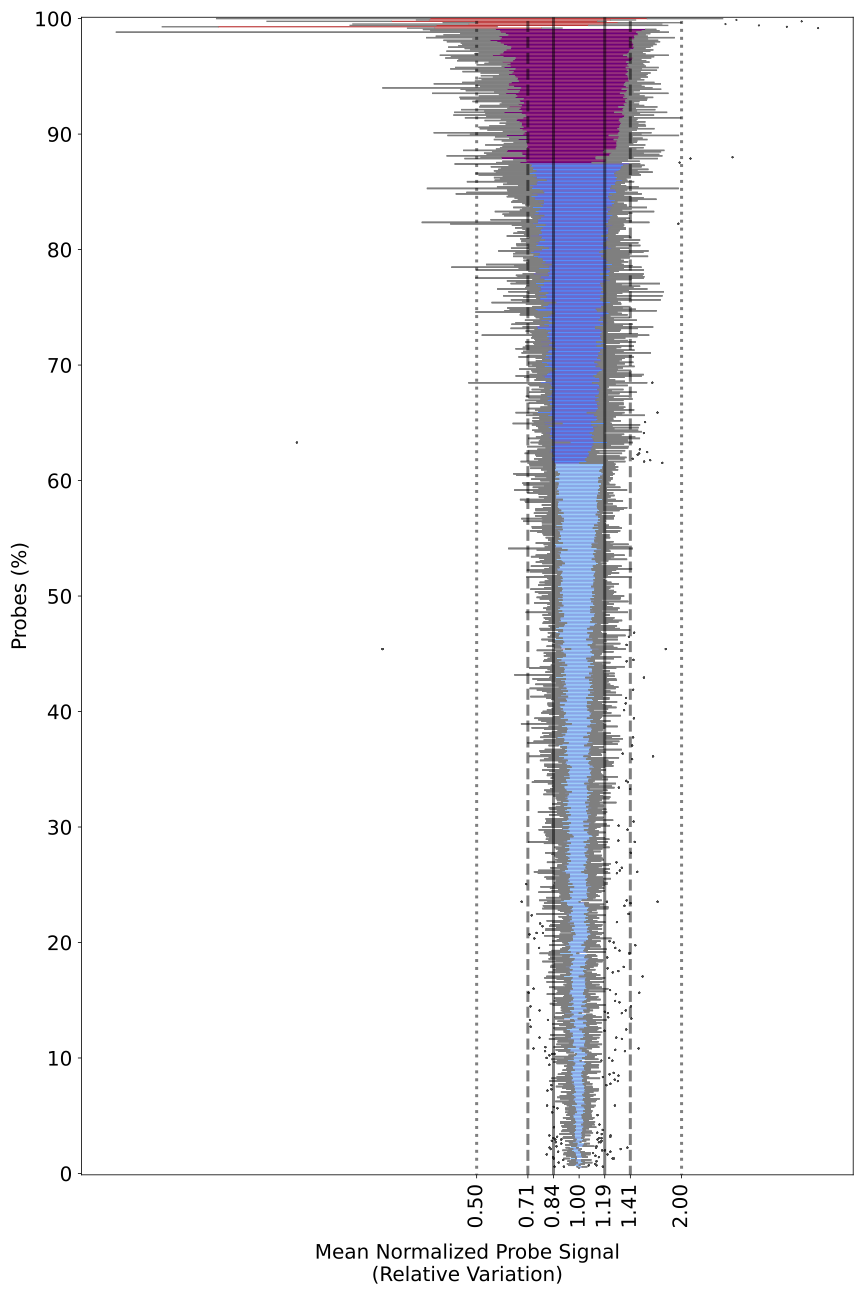
The circular ssDNA molecules containing the Nicking Loops are either achieved by targeting in the Bridge Capture™ (see FAQ interlinks below) or with non-targeted Nicking Loop™ conversion & indexing. These serve as a template for the linear Rolling Circle Amplification (RCA) reaction (Figure 1 step 1). RCA produces a long concatemeric molecule containing multiple adjacent linear copies of the original circular template (see FAQ interlinks below).
A long linear molecule generated by RCA contains sequence structures that facilitate the formation of local hairpin loops at fixed positions (Figure 1 step 2). Each segment, spanning from the complete loop structure to the start of the next adjacent loop, represents one full cycle around the circular template. The double-stranded stem of each loop includes a recognition site for a specific nicking endonuclease enzyme, able to cleave only one strand of the stem sequence. This targeted digestion acombanied with mild heating cuts the long concatemeric molecule into multiple pre-defined DNA fragments, each representing a linear copy of the original circular template (Figure 1 step 3). The ends of these fragments contain complementary sequence motifs, enabling them to self-anneal. With the addition of a ligase, the fragments can be re-circularized into complementary copies of the original template (Figure 1 step 4). These circular molecules can serve either as templates for further amplification cycles or as ready-to-use sequencing libraries with circular sequencing platforms (see FAQ interlinks below).
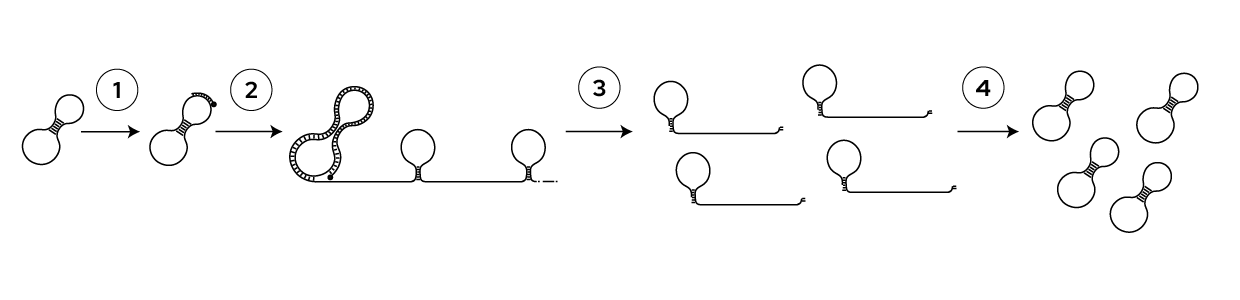
No.
Both the Bridge Capture™ and the Nicking Loop™ workflows can be completed using standard molecular laboratory equipment such as pipettes, vortexes, spinners, and PCR instrument. No specialized or uncommon equipment is necessary to successfully carry out the protocol.
Yes.
Bridge Capture™ can analyze variety of the different sample materials. Routinely tested sample types are cfDNA, gDNA, and cDNA. The technology has demonstrated promising results on direct targeting of RNA, as well as capturing unpurified DNA from urine and saliva.
For Nicking Loop™ we have tested gDNA during the workflow set up.
No.
Bridge Capture™ is compatible with all NGS platforms on the market. In addition to Illumina platforms, Bridge Capture™ workflow has been successfully completed with Ion Torrent and Oxford Nanopore NGS platforms. Nicking Loop™ has been successfully demonstrated with Illumina platforms. See FAQ interlinks below for an overview of how the technology outputs fit to different sequencing platforms.
Yes.
Over the past decade, advances in sequencing technologies, competition among sequencing providers, and increased demand and investments have led to a significant decrease in the cost of DNA sequencing. This has enabled, among other things, affordable whole-genome and whole-exome sequencing – applications where the sequencing depth requirement is moderate.
Liquid biopsies, on the other hand, are based on detection of rare somatic variants in cell-free DNA, the detection of which requires very deep sequencing. Performing whole-genome or whole-exome sequencing at such sequencing depths will remain unfeasible, irrespective of how much the sequencing costs decrease.
Bridge Capture™ provides various advantages when compared to different liquid biopsy techniques. We therefore provide three separate answers for this question where Bridge Capture™ is compared to amplicon-based techniques (see FAQ interlinks below), hybrid capture-based techniques (see FAQ interlinks below) and molecular inversion probe-based techniques (see FAQ interlinks below).
One key benefit Bridge Capture™ has over all of these techniques is the capability to pool the samples directly after the targeting step without quantification. This approach ensures that the workload does not increase linearly with the number of samples, offering a distinct advantage in scalability (see FAQ interlinks below).
There are multiple optional outputs for Bridge Capture™ and Nicking Loop™.
The key output of both technologies is the circular library, from which the other outputs are derived if needed. The circular library can be achieved in multiple ways with Bridge Capture™ or Nicking Loop™. The circular library can be directly sequenced with a circular DNA -based sequencing technology, such as Complete Genomics’s DNBSEQ , Element’s Aviti and PacBio’s Onso. Prior to sequencing the circular library can also be selectively accessed or unwanted parts of it can be deleted and still retain the original circular library for future use due to the possibility of concurrent circular DNA amplifications.
Optionally, the circular library can be processed further by amplifying it with RCA (see FAQ interlinks below), making the newly formed ssDNA concatemer directly adaptable to long-read sequencing platforms, such as Oxford Nanopore Technologies.
From the amplified ssDNA concatemer the individual monomers can be amplified with a limited cycle indexing PCR (see FAQ interlinks below). The amplicons are traditional linear libraries which can be sequenced with short-read platforms like Illumina and Ion Torrent.
Bridge Capture™ is a highly sensitive molecular quantification technique. Its typical oncological use cases involve quantification of mutations (including SNVs, indels and gene fusions) and CNVs (see FAQ interlinks below). One use case is mutation/CNV detection from tumor DNA. Another use case is mutation/CNV detection from cfDNA extracted from patient’s blood plasma, which has applications in Tx and MRD.
Probe design requires detailed information of target DNA sequence or genomic coordinates of the mutation.
A panel is a subset of the probe library.
In context of cancer diagnostics, a panel is a predefined set of probes to target specific regions of the genome to detect genetic mutations, variations or other alterations associated with a particular cancer type.
A probe is a DNA oligomer that binds to complementary DNA sequence. Bridge Capture™ uses probe pairs which are bound together by a third, non-target-binding oligomer (See Figure 1). The first enzyme reaction called GapFill takes place after the probe hybridizes to its target (see FAQ interlinks below).
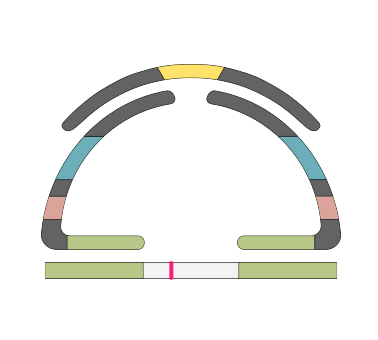
The probe library contains all the probes Genomill has developed.
Probe mix is a solution containing specific probes, or a physical embodiment of a panel it represents. Probe mix is an essential part of the Bridge Capture™ chemistry.
UMIs are sequences associated with each read that permit identifying the original molecule from which amplification took off. UMIs permit accurate target quantification as well as error correction. UMIs are introduced by incorporating random nucleotides into predefined regions of the probes. When the probes are sequenced, the UMI sequences are also read. The UMIs are designed to be long enough to create sufficient variation, so that each probe hybridization event to the target material will have a high probability of having its own unique UMI. This high level of variation is crucial for accurate quantification, as it ensures that each individual molecule is accurately identified and counted. This permits even rare variants to be quantified with high accuracy.
Bridge Capture™ process is illustrated in Figure 1 and the technology is illustrated in detail in Figure 8.
Bridge Capture™ is an efficient targeted pre-sequencing technology which offers a rapid, highly sensitive, platform agnostic and cost-effective molecular quantification solution.
In Bridge Capture™ the sample is targeted with Bridge Capture™ probes. A successful targeting of a specific sequence requires simultaneous binding of two probes, which are held together by a bridge oligo. Both probes must bind to their respective target sequences for the Bridge Capture™ to be successful. To ensure accurate identification of unique binding events, UMIs (see FAQ interlinks below) are included in the probes.
Once the probes have found their intended targets, the gap between probes is copied from the target sequence and ligated to form gap-filled circular probe construct, which can be optionally sequenced directly with a circular-based sequencer like Complete Genomic’s DNBSEQ, Element Aviti or PacBio Onso. This circular construct is linearly amplified by RCA to generate multiple copies of the circular probe, which can be optionally sequenced with long-read sequencing platforms, such as Oxford Nanopore Technologies.
After linear amplification, sequencing platform-specific adapters are introduced through a limited-cycle indexing PCR.
To ensure optimal quality and purity, the resulting libraries are purified with bead purification protocols. This step helps to remove any impurities and contaminants that may affect the accuracy of the sequencing data.
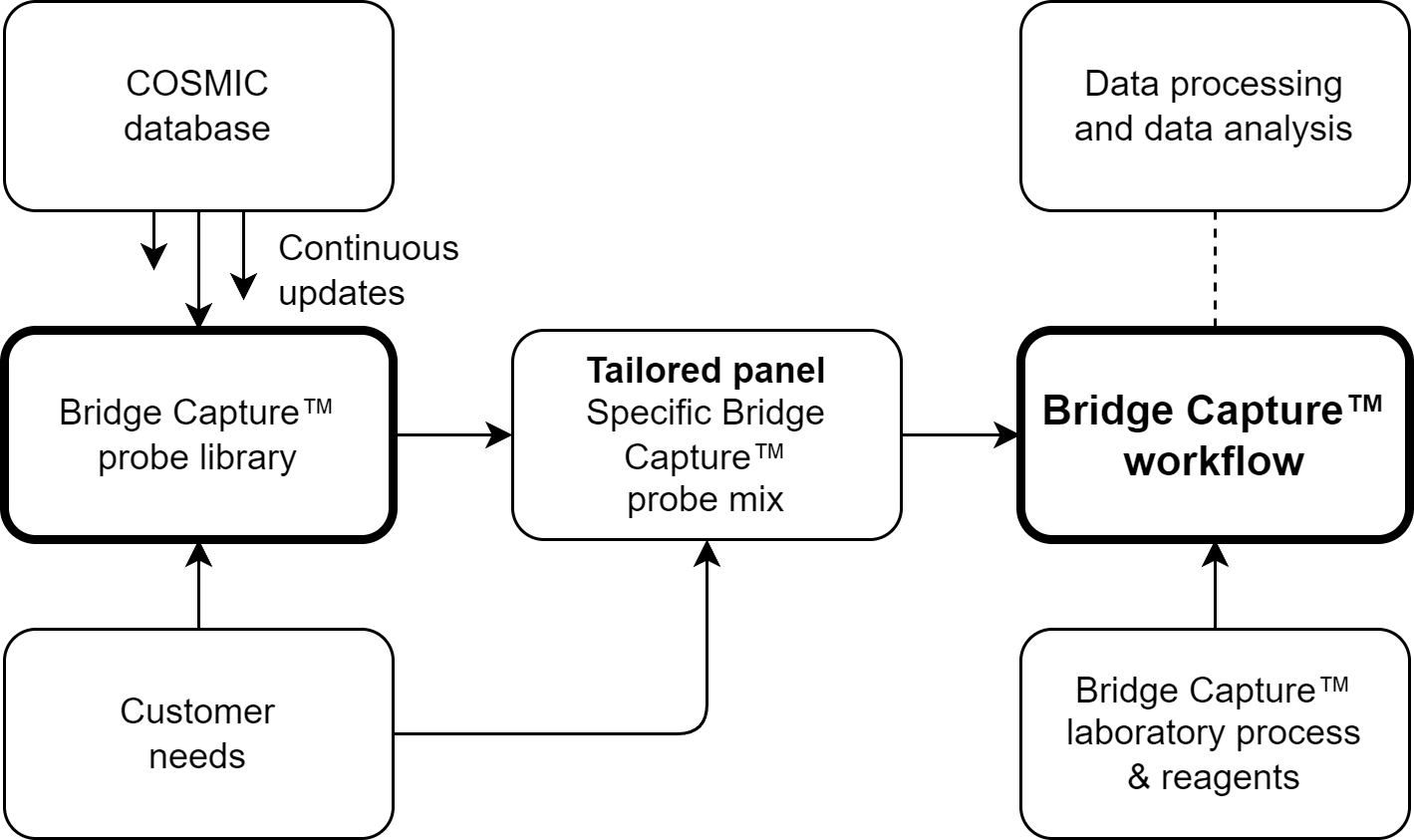
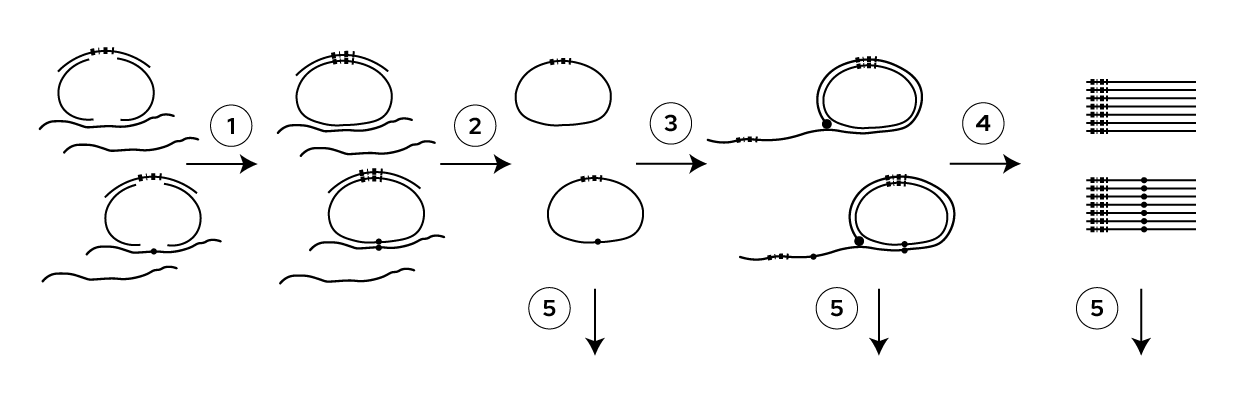
The base pair size of the libraries varies depending on the probe design. The length is usually approximately 280 base pairs.
The length of the libraries varies depending on the probe design. The length is usually approximately 225 bases.
Bridge Capture™ is a patented laboratory process workflow. The bridge structure connects the two target-specific probes, enabling the capture of the target region and its subsequent linear amplification. This innovative design allows the targeted region to be copied to the probe gap. This permits capture of relatively long target regions while keeping the cost of probe synthesis down by using shorter individual oligos. Bridge is the upmost oligo in the structure in Figure 1.
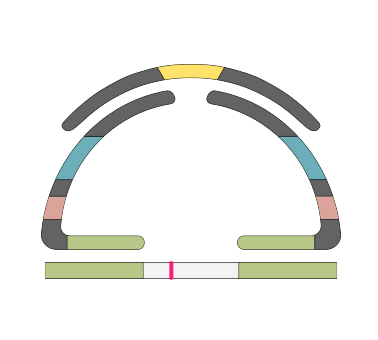
Our clinical testing has not yet been sufficient to establish these numbers. As of 2024-11, we are collecting further sample cohorts to establish numbers for type I (false positive) and type II errors (false negative).
The Bridge Capture™ workflow is designed to be fast and efficient, taking less than 5 hours to complete with only 5 minutes of hands-on time required, compared to 7 hours on amplicon-based workflows or at least 8 hours on hybrid capture-based workflows. This streamlined workflow allows for rapid sample processing, saving valuable time and resources (<See Figure 1>).
Once the workflow is complete, the subsequent NGS analysis duration depends on the sequencing technology used.
Figure 1 Duration breakdown of Bridge Capture™ Tx and MRD laboratory workflows.
The main factors that impact the sensitivity of the Bridge Capture™ are sample quality and total amount of DNA present. It is worth noting that the sensitivity varies slightly between different probes, which is most likely due to the unique binding characteristics determined by the probe and target sequences. In our publication (Adamusová et al. 2024), we observed a sensitivity range of 0.03% to 0.2% MAF, which depends on the specific probe. These MAFs were determined through serial dilutions of fragmented tumor biopsy DNA to fragmented gDNA, which were subsequently sequenced on the NovaSeq 6000. We can optimize the performance characteristics of any probes based on customer preferences.
The cost of Bridge Capture™ consists of two parts: sample preparation and sequencing. The sample preparation cost is around USD 10 per sample regardless of the panel size with the reagent rates available to us. We expect the cost to come down with further optimizations. The approximated sequencing costs are presented in the Table 1 below.
The low sample preparation cost is based on the simplicity of the protocol, as well as the small amounts of low-cost reagents (see FAQ interlinks below). The low sequencing cost is based on the efficient targeting of the sequencing effort as well as the faithful representation of the read counts in the sample, provided by the linear signal amplification. Cost of labor is not included in the estimation.